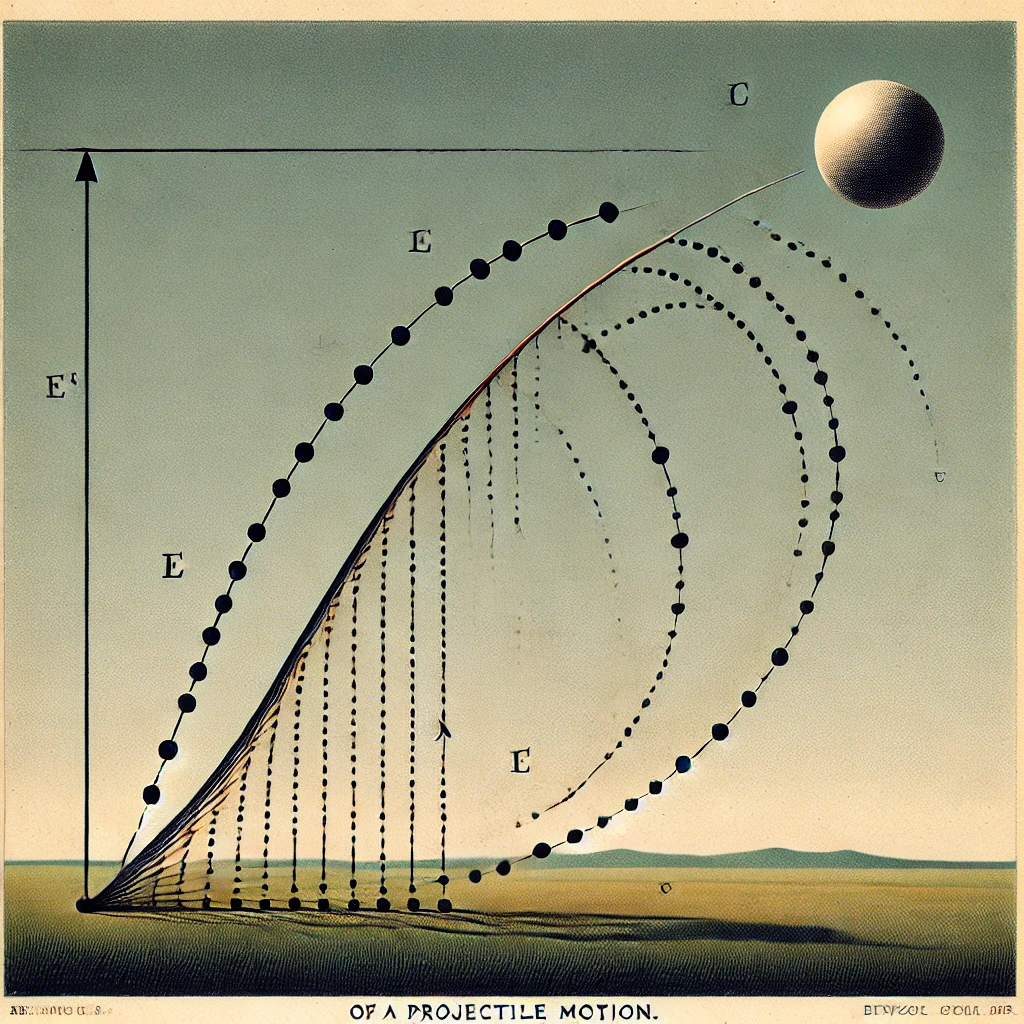
Introduction to Projectile Motion
Projectile motion is a significant concept in physics that describes the motion of an object that is thrown, kicked, or otherwise propelled into the air and is subject only to the force of gravity and air resistance. Unlike linear motion, where an object moves in a straight line, projectile motion involves a curved trajectory. The path that a projectile follows is called its trajectory, which can often be described as a parabola due to the effects of gravitational acceleration pulling the object downwards.
In order to understand projectile motion fully, it is essential to define what constitutes a projectile. A projectile is any object that is thrown or propelled and can travel through the air until the force of gravity brings it to the ground. Examples of projectiles include balls, arrows, and even large objects like rockets during their flight phase. Unlike static or uniform linear motion situations, in projectile motion, the variables change dynamically, allowing for complex behaviors that can be predicted using kinematic equations.
One of the primary characteristics of projectile motion is that it can be analyzed as the combination of two independent motions: horizontal and vertical. While the horizontal component of motion occurs at a constant velocity (assuming negligible air resistance), the vertical component is influenced by the acceleration due to gravity, which is approximately 9.81 m/s² downward. Consequently, as a projectile moves through the air, it rises, reaches a peak altitude, and subsequently descends, illustrating a symmetrical parabolic path.
Understanding these fundamental aspects of projectile motion is crucial for not only physics but also various real-world applications, such as sports, engineering, and space exploration. By grasping the principles of how projectiles move, we can predict their future positions at any point during their flight, leading to a more comprehensive appreciation of motion dynamics in nature.
Key Concepts of Projectile Motion
Projectile motion is a fascinating subject in the realm of physics, characterized by the unique interaction of two independent components: horizontal motion and vertical motion. Understanding these elements is essential for grasping how objects move through space when projected. The independence of these components is a cornerstone of projectile motion, allowing physicists to analyze them separately while maintaining an integrative approach to their combined behavior.
Horizontal motion in projectile motion occurs at a constant velocity. This is because, in the absence of external forces like air resistance, there are no horizontal accelerations acting on the projectile once it has been launched. An object remains in uniform motion along the horizontal axis, showcasing a fundamental principle of Newton’s First Law of Motion. Therefore, the distance traveled horizontally can be calculated using the formula: distance equals velocity multiplied by time.
On the other hand, vertical motion is influenced predominantly by gravity, which acts as a constant acceleration of approximately 9.81 m/s² downwards. When an object is projected upwards, its vertical velocity decreases until it momentarily stops at its peak height. Subsequently, it accelerates downward, gaining speed until it reaches the ground. This behavior can be described through the equations of motion, specifically taking into account the initial velocity, time, and displacement.
The interplay between these two motions results in a parabolic trajectory, which is the hallmark of a projectile’s path. The curvature of this trajectory can be attributed to the continuous effect of gravity on the vertical component, while the horizontal component maintains a constant rate of motion. Thus, a thorough understanding of the independence and principles governing horizontal and vertical motions lays the groundwork for comprehending the complexities of projectile motion.
The Parabolic Path Explained
Projectile motion, a fundamental concept in physics, dictates that objects follow a parabolic trajectory when influenced solely by gravity and initial launch conditions. Unlike linear motion, which is straightforward, the curved path of a projectile arises from the simultaneous effects of horizontal and vertical forces. The motion can be broken down into two components: horizontal motion, which is constant, and vertical motion, which is affected by gravitational acceleration.
The mathematics underpinning the parabolic trajectory stem from the equations of motion. The position of a projectile can be described using quadratic equations, where the path takes the form of a parabola. This relationship is evident when the vertical position is plotted against horizontal distance, illustrating the characteristic “U” shape. Initially, when a projectile is launched, it ascends, due to its initial velocity, until it reaches its peak height. Beyond this apex, gravity causes the projectile to descend, tracing the curved path typical of parabolic motion.
Moreover, the angle of projection plays a crucial role in determining the trajectory. An object launched at a higher angle will achieve greater vertical height before descending, thus elongating the parabolic arc. Conversely, a smaller launch angle results in a flatter trajectory, influencing both range and height. Mathematically, optimal angles for maximum distance generally stabilize around 45 degrees in ideal conditions, though factors such as air resistance can affect real-world outcomes.
In addition to the initial velocity and launch angle, the uniform acceleration due to gravity (approximately 9.81 m/s²) ensures that the projectile will always follow a specific curved path, regardless of its initial conditions. Understanding these principles enables accurate predictions of a projectile’s motion, allowing applications ranging from sports to engineering to utilize these concepts effectively.
Factors Influencing Projectile Motion
Projectile motion is a crucial concept in physics, characterized by the curved trajectory of an object in the air after being projected. Several factors influence this type of motion, including the angle of projection, initial velocity, air resistance, and the height from which the projectile is launched. Understanding these elements is essential for both theoretical studies and practical applications.
The angle of projection is one of the primary factors affecting the trajectory of a projectile. When an object is launched, the angle at which it is projected determines the shape of its path and influences both the maximum height reached and the distance traveled. A projection angle of 45 degrees typically yields the maximum range in a vacuum, assuming no other forces act on the projectile. However, varying the angle can either increase or decrease the vertical height and horizontal distance depending on the specific circumstances.
Initial velocity also plays a significant role in projectile motion. The greater the speed at which a projectile is launched, the further it travels and the higher it ascends, assuming all other factors remain constant. Real-life examples, such as a basketball shot or a cannon firing, illustrate how varying the initial velocity impacts the outcome of the projectile’s flight.
Air resistance is another critical factor that alters projectile motion. This force, which opposes the motion of the object, becomes particularly significant at higher velocities. For instance, a feather and a typical stone, when dropped from the same height, will not follow the same trajectory due to the greater effects of drag on the feather. Lastly, the height from which a projectile is launched can affect both its time of flight and range, as launching from an elevated position often leads to increased distances covered. By analyzing these factors, we can gain insights into the complex nature of projectile motion and its practical applications in sports, engineering, and beyond.
Applications of Projectile Motion
Projectile motion plays a crucial role in numerous fields, particularly in sports, engineering, and space exploration. Understanding the principles of projectile motion enables athletes to enhance their performance, engineers to design effective projectiles, and scientists to launch satellites successfully into orbit. Each of these domains relies on the same fundamental physics that governs the path of a projectile, highlighting the widespread relevance of this topic.
In the realm of sports, the trajectory of a basketball shot is a prime example of projectile motion in action. Players must consider factors such as angle, force, and the Earth’s gravitational pull when attempting a basket. A well-calculated shooting angle increases the likelihood of success, as the ball follows a curved path dictated by its initial velocity and the forces acting upon it. Coaches often study and analyze these trajectories to optimize training techniques and improve overall team performance.
Similarly, engineering applications of projectile motion are vast. Designers of weapons, rockets, and various projectiles must meticulously calculate trajectories to ensure accuracy and effectiveness. By applying principles of physics, engineers can predict how projectiles will behave under different conditions, including air resistance and gravitational effects. This knowledge is vital for refining designs, assessing performance, and ensuring safety in their deployment.
In the field of space exploration, the calculations involved in launching satellites into orbit heavily draw from the principles of projectile motion. Engineers must carefully determine the launch angle and velocity needed to overcome Earth’s gravity and achieve a stable orbit. These calculations are fundamental to ensuring that satellites can carry out their intended functions, such as communication, weather monitoring, and Earth observation, thereby enhancing our understanding of the planet.
Overall, the applications of projectile motion are integral to advancements in sports, engineering, and space exploration, demonstrating its significance across diverse fields.
Mathematical Framework of Projectile Motion
Projectile motion is governed by a specific set of mathematical principles which enable the calculation of vital aspects such as range, height, and time of flight. One fundamental formula used to determine the range of a projectile is given by R = (v0² sin(2θ)) / g, where R denotes the range, v0} is the initial velocity, θ is the launch angle, and g represents the acceleration due to gravity (approximately 9.81 m/s²).
To illustrate how this formula is applied, consider a projectile launched with an initial velocity of 20 m/s at an angle of 30 degrees. By substituting these values into the range equation, we convert the angle into radians and perform the calculations. The result reveals that the projectile travels a specific distance before returning to the ground.
Next, for calculating the maximum height reached during projectile motion, we utilize the formula H = (v0² sin²(θ)) / (2g). If we apply the same initial velocity of 20 m/s and the angle of 30 degrees, the maximum height can be calculated, revealing the peak point of the trajectory. This value is essential for comprehending how far upward the projectile travels before descending.
Additionally, the total time of flight can be determined using the formula T = (2v0sin(θ)) / g. This equation allows us to estimate how long the projectile remains airborne after being launched. In practice, these calculations can inform a variety of real-world applications, including sports, engineering, and physics education.
Each of these equations showcases the intricate relationship between the angles, velocities, and gravitational forces that characterize projectile motion, thus providing a comprehensive understanding of its dynamics.
Conclusion
Understanding projectile motion is essential for various fields, ranging from physics education to engineering applications. At its core, projectile motion involves the trajectory of an object launched into the air, characterized by a parabolic path influenced by gravitational and initial velocity factors. This fundamental concept provides valuable insights into the behavior of moving objects, allowing students to grasp key principles of motion and acceleration. The learning of projectile motion is not merely an academic exercise; it serves as a foundation for further studies in mechanics and dynamics.
For students, comprehending the nuances of projectile motion aids in developing critical thinking and problem-solving skills. It allows them to apply mathematical calculations to real-world scenarios, such as calculating the optimal launch angle for achieving maximum range or determining the time an object remains airborne. This mastery is paramount for those pursuing careers in the physical sciences, where understanding motion is necessary for experimenting and validating theoretical models.
In the realm of engineering, knowledge of projectile motion is equally significant. Engineers significantly rely on these principles when designing structures, launching projectiles, or analyzing the paths of various objects under gravitational influence. From fireworks to sports equipment, the application of projectile motion principles has real-world implications. Recognizing the importance of this concept enhances engineers’ ability to innovate and resolve complex challenges effectively.
Moreover, understanding projectile motion promotes a deeper appreciation of the physical world. It encourages individuals to observe and analyze the dynamics of everyday objects, contributing to a more scientifically literate society. Thus, grasping the fundamentals of projectile motion is vital for students, engineers, and anyone interested in engaging meaningfully with the sciences, ensuring that they are well-equipped to explore the myriad possibilities that lie within this fascinating aspect of physics.