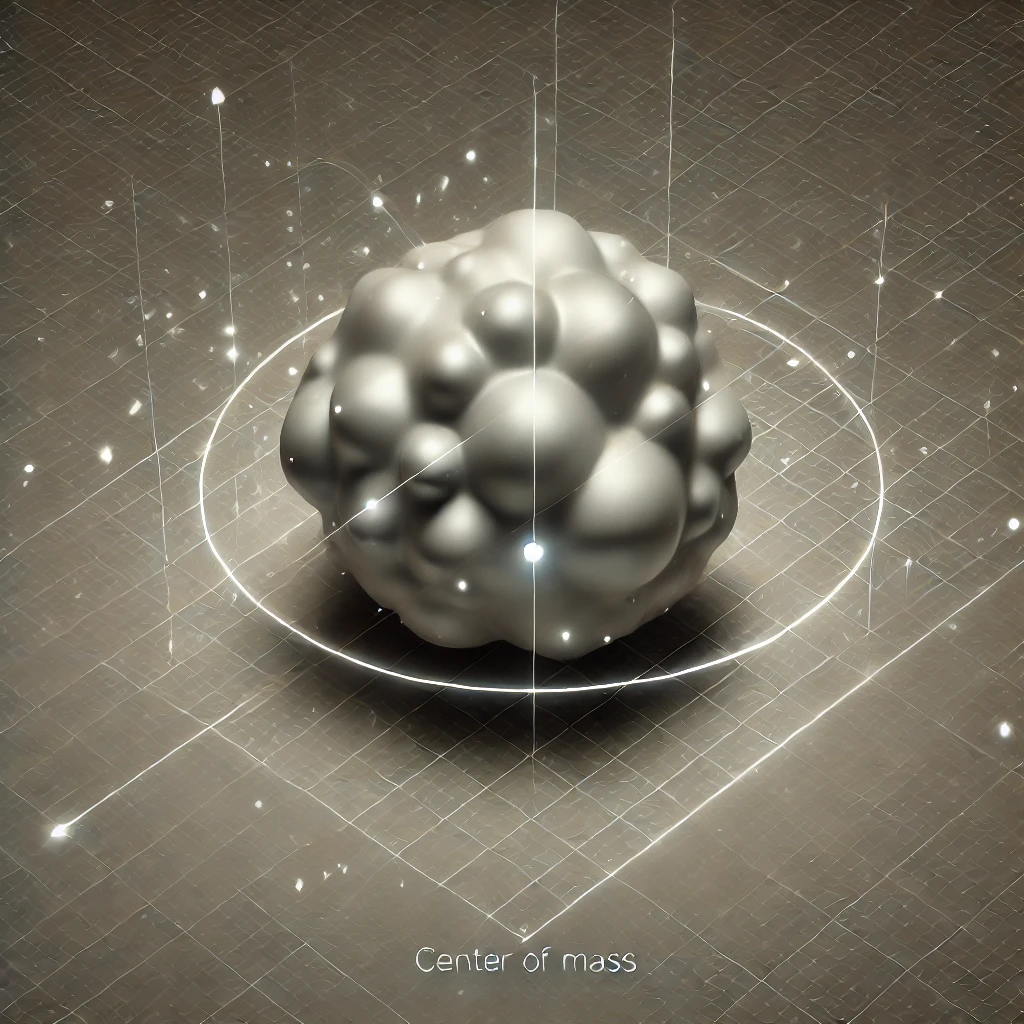
Introduction to Center of Mass
The center of mass is a fundamental concept in the fields of physics and engineering, serving as a critical point that defines the distribution of mass within a body or system of particles. Essentially, it represents the average location of all the mass in an object, providing insight into the object’s balance and stability. Understanding the center of mass is invaluable for analyzing the motion of various physical bodies, including rigid objects, fluids, and even celestial bodies in space.
It is important to distinguish between the center of mass and the center of gravity, even though these terms are often used interchangeably. The center of gravity refers specifically to the point at which the gravitational force acts on an object. In uniform gravitational fields, the center of mass and the center of gravity coincide. However, in non-uniform gravitational fields, or when the mass distribution is irregular, these points may differ significantly. This differentiation is crucial for accurate calculations in fields such as aerospace engineering, where the behavior of spacecraft in varying gravitational forces is of prime importance.
Grasping the concept of center of mass is essential not only for theoretical purposes but also for practical applications. For example, engineers must account for the center of mass when designing structures to ensure they can withstand various forces and remain stable during use. Likewise, physicists use this concept to predict the motion of objects under the influence of different forces, thereby enhancing our understanding of physical laws. The analysis of the center of mass also extends to dynamics, where it plays a pivotal role in discussions of momentum and energy conservation.
Definition and Mathematical Representation
The center of mass (COM) is a key concept in physics and engineering, representing the average position of an object’s mass distribution. It helps describe how mass is spatially arranged in a system and plays a critical role in understanding motion and stability.
Discrete Systems:
For a system consisting of discrete particles, the center of mass R is calculated using the formula:
Where:
- M is the total mass of the system.
is the mass of the i-th particle and
is the position vector of the i-th particle.
This equation essentially calculates a weighted average of the particle positions, where the weights are their masses. The center of mass represents the point at which the entire mass of the system can be considered concentrated for the purposes of translational motion analysis.
Continuous Systems:
For objects with continuous mass distributions, where mass is spread out rather than concentrated at specific points, the center of mass is found using integrals. The formula is given by:
Where:
- M is the total mass of the object.
- dm represents an infinitesimal mass element.
- r is the position vector of the mass element.
In this case, the integral sums the contribution of each infinitesimal mass element across the entire object. This approach is useful for continuous objects, such as rods, plates, or spheres, and can be computed using different coordinate systems (e.g., Cartesian, polar, spherical) depending on the object’s geometry.
For example, in the case of a uniform object of length and mass M, the center of mass would lie at the geometric center, which simplifies the integral.
Coordinate Systems:
Selecting the right coordinate system (e.g., Cartesian, cylindrical, or spherical coordinates) based on the symmetry and geometry of the object can simplify these calculations. By choosing the most appropriate system, both the integral and the result become easier to manage and interpret.
Applications:
The concept of the center of mass is widely used in various fields such as:
- Dynamics: To analyze the motion of bodies in terms of translational and rotational motion.
- Stability analysis: To predict how objects behave under external forces.
- Structural engineering: To determine load distribution and support stability.
Utilizing appropriate coordinate systems—such as Cartesian or polar coordinates—can significantly simplify these calculations, enhancing both accuracy and ease of application. Therefore, understanding the mathematical representation of the center of mass is essential for various applications in physics, including dynamics, stability analysis, and structural engineering.
Factors Affecting the Center of Mass
The center of mass of an object is a critical point that reflects the distribution of mass within that object. Several factors can significantly influence the position of the center of mass, including mass distribution, shape, and configuration of the particles that constitute the object. Understanding these factors enhances insights into both static and dynamic systems where the center of mass plays an essential role in motion mechanics.
One of the primary determinants of the center of mass is the distribution of mass throughout the object. When mass is unevenly distributed, the center of mass shifts towards the denser concentration. For instance, if a rigid body has more mass concentrated at one end, its center of mass will be located closer to that denser section. This phenomenon occurs in various practical applications, such as in sports equipment design, where weight distribution can affect performance and maneuverability.
The shape of the object also plays a critical role in determining the location of the center of mass. Objects with symmetrical shapes, such as spheres and cubes, tend to have their center of mass positioned at the geometric center. In contrast, irregularly shaped objects may have a more complex mass distribution, causing their center of mass to be located away from the geometric center. This shift can be crucial in engineering and construction, as the structural integrity and stability of an object are often analyzed based on this central point.
Lastly, the arrangement or configuration of the particles within a system can further modify the center of mass. For example, in a multi-particle system, moving particles closer together or dispersing them can change the overall center of mass. Understanding these concepts enables scientists and engineers to predict the behaviors of various systems under force, which is invaluable when analyzing the dynamics of objects in motion.
Center of Mass in Different Geometries
The center of mass is a fundamental concept in physics that represents the average position of all the mass in a given object. When analyzing various geometric shapes, determining the center of mass can simplify calculations in mechanics and provide insights into stability and balance. Each geometric shape exhibits unique characteristics regarding the computation of its center of mass.
For symmetrical shapes, the center of mass is often located at a point that aligns with the geometric center. For instance, in a sphere, the center of mass can be found at its geometric center. This is because the mass is evenly distributed in all directions from that point. Similarly, in cubes and rectangular solids, the center of mass lies at the intersection of the diagonals within the shape, which corresponds to the centroids of the individual axes.
In more linear objects such as rods, the center of mass is found at the midpoint if the rod has uniform density. This property is vital for understanding how such simple shapes behave under various forces. When these objects are subjected to external forces, the behavior of the entire system can often be predicted by focusing on the center of mass.
However, irregular shapes challenge this straightforward approach. For such objects, the center of mass must be determined using more complex methods, such as integration or the use of physical balancing techniques. For example, one can physically balance the object on a point until equilibrium is achieved, which will indicate the location of the center of mass. These methods require careful consideration, as the distribution of mass significantly influences the stability and dynamics of these bodies.
In various applications ranging from engineering to robotics, an accurate understanding of the center of mass allows for better structural design and improved motion analysis. By mastering the principles of determining the center of mass across different geometries, one gains a valuable tool that aids in a wide range of scientific and engineering disciplines.
Applications of Center of Mass in Real Life
The concept of center of mass plays a crucial role in various real-world applications, spanning across diverse fields such as vehicle dynamics, sports, robotics, and structural engineering. Its significance lies in understanding how mass distribution affects stability and performance, contributing to enhanced design and functionality.
In vehicle dynamics, the center of mass is pivotal in determining how a vehicle behaves during maneuvers. A lower center of mass generally improves stability, reducing the likelihood of rollover accidents. Manufacturers strategically design vehicles to optimize the center of mass placement, balancing weight distribution between the front and rear axles. This optimization enhances handling characteristics and contributes to overall safety while driving.
In the realm of sports, athletes leverage their understanding of center of mass to enhance performance. For instance, gymnasts expertly manipulate their body position during routines, effectively shifting their center of mass to maintain balance and control during complex maneuvers. Similarly, in sports like high jump or pole vault, athletes aim to position their center of mass above the bar, creating an advantage during their jump. This awareness is integral to achieving optimal performance and minimizing the risk of injury.
Robotics also heavily depends on the concept of center of mass. Engineers consider the center of mass when designing robots to ensure stability during movement. Autonomous robots with a low center of mass can navigate uneven terrains more effectively, improving their operational efficiency. Adjusting the position of components within the robot can lead to better balance and enhanced agility, which are critical in competitive robotic applications.
Lastly, in structural engineering, the center of mass influences the design of buildings and bridges. Engineers must account for the distribution of mass within a structure to ensure it can withstand various forces, such as wind and seismic activity. By understanding how mass is distributed, they can enhance structural integrity and safety, thereby reducing the risk of catastrophic failure.
Through these examples, it is evident that the understanding of the center of mass is integral to various disciplines, significantly impacting design, stability, and performance in real-life applications.
Experiments and Demonstrations
The concept of center of mass can be better understood through a variety of hands-on experiments and demonstrations. These activities can be performed in a classroom setting or at home, making them accessible for learners of all ages. Conducting such experiments not only reinforces theoretical knowledge but also provides tangible experiences that enhance understanding.
One simple yet effective experiment involves using a ruler and a small weight, such as a washer or a marble. To visualize the center of mass, balance the ruler on your fingertip. The ruler will naturally find its center of mass, indicating the point where it can be balanced. By moving the weight along the length of the ruler, one can observe how the position of the center of mass changes. This demonstration illustrates how the distribution of mass affects balance and stability.
Another engaging activity includes creating a simple mobile using lightweight materials like straws and paper. By creating shapes with varying mass distributions, one can observe how the center of mass influences the equilibrium of the mobile. When balanced correctly, the mobile will remain stable, reflecting the core principle of center of mass in a visually appealing manner. This experiment encourages creativity while highlighting the fundamental physics involved.
A more advanced demonstration involves using a pendulum system. By attaching weights at different points along a string, one can see how the center of mass shifts with changes in the pendulum’s configuration. Analyzing the motion of the pendulum can help solidify the understanding of the center of mass in dynamic systems.
These simple experiments serve as excellent tools for visualizing and measuring the center of mass in various objects. Engaging in these hands-on activities can significantly enhance one’s grasp of the concept, making it a memorable learning experience.
Conclusion and Future Directions
In summary, the concept of the center of mass is pivotal in the realms of physics and engineering. It serves as a fundamental principle that aids in understanding the behavior of objects in motion, from the simplest systems to complex structures. The center of mass provides essential information regarding stability, equilibrium, and movement, enabling engineers and scientists to predict how objects will interact under various forces. As we explore the importance of the center of mass, it becomes evident that its applications extend beyond theoretical constructs, influencing practical designs in fields such as robotics, aerospace, and biomechanics.
As technology continues to evolve, there are numerous areas for further research and exploration relating to the center of mass. For instance, the integration of advanced computational tools and simulations in studying the center of mass can significantly enhance design accuracy. Investigating the center of mass in non-traditional materials, such as biomaterials and composites, also presents new opportunities for innovation. Moreover, ongoing studies in related fields, such as fluid dynamics and particle physics, will likely yield insights that refine our understanding of how centers of mass operate under varying physical conditions.
Furthermore, with the rise of autonomous systems and artificial intelligence, understanding the center of mass can play a crucial role in ensuring the stability and functionality of these technologies. Enhancements in motion capture and modeling can lead to improved navigation and manipulation capabilities in robotic systems. Addressing the challenges associated with dynamic environments will require continued research into the principles governing the center of mass, ultimately leading to advancements in safety and efficiency across multiple applications.
The ongoing exploration of the center of mass holds great potential for innovation. By bridging theoretical knowledge with practical applications, we can unlock new possibilities that will shape the future of science and technology.